DESIGN OF HIGH RHENIUM CONTAINING SINGLE CRYSTAL SUPER-ALLOYS
WITH BALANCED INTERMEDIATE AND HIGH TEMPERATURE CREEP STRENGTHS
T.Kobayashi, Y.Koizumi, S.Nakazawa, T.Yamagata and H.Harada *
* High Temperature Materials Research Group
National Research Institute for Metals, Tsukuba Science City, Japan.
Published in Proceedings of the Fourth International Charles Parsons Turbine Conference on
"Advances in Turbine Materials, Design and Manufacturing", held at Newcastle upon Tyne, UK,
4-6 November 1997, pp. 766-773. The Institute of Materials.
ABSTRACT
- A single crystal (SC) superalloy with balanced intermediate and high temperature creep strengths was designed by using NRIM Alloy Design Program (ADP) with help of Cluster Variation Method (CVM) computer program based on statistical thermo-dynamics. An alloy TMS-75 with a multi chemical composition, having 3wt% Cr, 5wt% Re, etc, was selected and tested in terms of microstructural stability, creep strength, hot corrosion resistance and so on. The creep test showed that the alloy has a good balance of creep strength over the 900-1150$B!n(B temperature range; typical rupture lives at 900$B!n(B-392MPa and 1040$B!n(B-137MPa being 961 h and 1526 h, respectively. A laboratory scale hot corrosion test showed a very good hot corrosion resistance as well. An Ir addition to TMS-75 improved the phase stability.
INTRODUCTION
- The third generation single crystal (SC) superalloys with high Re contents have been paid much attention for their high creep strengths especially at intermediate temperatures, as well as the excellent hot corrosion resistance in spite of the low Cr contents [1, 2]. However, the problem still remains with the phase stability and its related degradation of mechanical properties. For instance, it is reported with CMSX-10 (RR3000), which is one of the third generation alloys, that the TCP phase tends to precipitate at higher temperature and longer exposure time conditions, which leads to a decrease in creep strength. Thus as shown in Figure 1, the improvement in temperature capability at higher temperature region is not significant compared with other SC superalloys.
- In the present study, a single crystal superalloy with balanced intermediate and high temperature creep strengths is designed by using our computer programs. The selected alloy is examined in terms of the solution treatment window, microstructural stability, creep strength, hot corrosion resistance, and so on. An iridium (Ir) addition was also tried with view to stabilizing the microstructure.
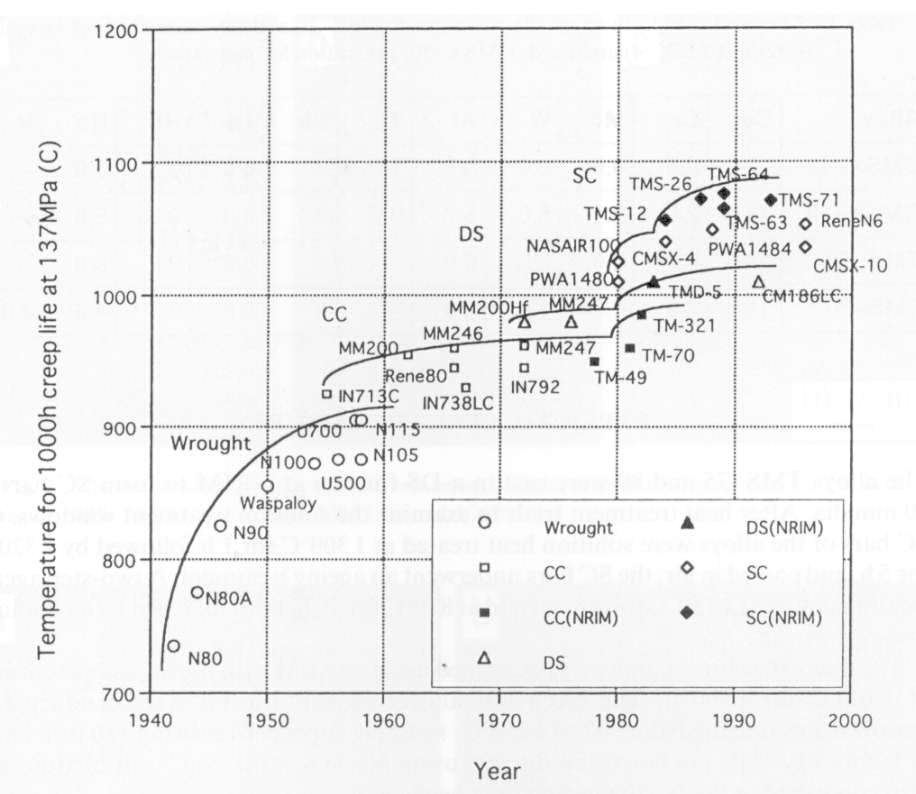
- Figure 1 The historical improvement in creep rupture temperature capability of Ni-base uperalloys. Capabilities of NRIM experimental alloys are also shown.
ALLOY DESIGN
- A single crystal (SC) superalloy with balanced intermediate and high temperature creep strengths was designed by using NRIM Alloy Design Program (ADP) [3, 4] with help of Cluster Variation Method (CVM) [5, 6] computer program based on statistical thermo-dynamics with using Lennard-Jones interatomic potentials.
- The design condition was as follows; alloy density to be smaller than 8.9, solution heat treatment window to be larger than 40$B!n(B, lattice misfit to be negative at high temperatures but not beyond certain extent, creep rupture lives both at 900$B!n(B-392MPa and at 1040$B!n(B-137MPa to be longer than 1000 h, etc. An alloy with a multi chemical composition, having 3wt% Cr, 5wt% Re, etc., was selected. An addition of 3wt% Ir was tried with view to stabilising the microstructure. The chemical compositions of the alloys, TMS-75 and TMS-80, are shown in Table 1.
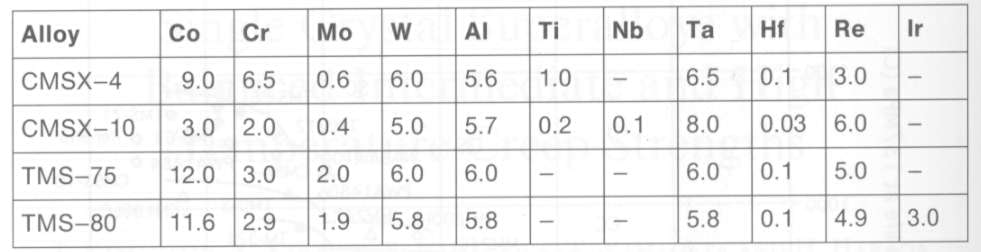
- Table 1 Chemical composition of alloys designed, TMS-75 and 80, with those of typical second (CMSX-4) and third (CMSX-10) generation SC superalloys.
EXPERIMENTAL PROCEDURE
- The alloys TMS-75 and 80 were cast in a DS furnace at NRIM to SC bars of 10 mm dia. After heat treatment trials to examine solution treatment windows, the SC bars of the alloys were solution heat treated at 1300$B!n(B for 1 h followed by 1320$B!n(B for 5 h, and cooled in air.
A two-step aging treatment, first at 1150$B!n(B for 4 h, second at 870$B!n(B for 20 h, both followed by air cooling, was performed.
- The microstructure stability was examined by using SEM with SC samples heated at 1100$B!n(B for 1000 h. The creep test and creep rupture test were conducted at temperatures ranging from 900 to 1150$B!n(B, resulting rupture lives being 150 to 1500 h. A laboratory scale hot corrosion dip test using Na2SO4-25%NaCl salt mixture was also conducted at 900$B!n(B, the testing time being 20 h.
RESULTS AND DISCUSSION
- Figure 2 shows the result of single step solution treatment trials at different temperatures for 2 h using TMS-75. At up to 1300$B!n(B(a), eutectic $B&C(B' remains undesolved but the amount is already small. The perfect solution is achieved at 1320$B!n(B(b) and at 1340$B!n(B. A very small amount of incipient melting starts to occur at 1360(c). From these observations, the predicted solution heat treatment window, 40$B!n(B, is found to be achieved with TMS-75. The same result was obtained with TMS-80. Considering that the heat treatment trials are single step heating up to the holding temperatures, the heat treatmentability of the designed alloys are exceptionally good as such high Re containing alloys. Thus the heat treatment of the creep samples were performed at 1320$B!n(B for 5 h, after heating at 1300$B!n(B for 1 h, which is a pseudo stress release treatment to avoid any recrystalisation formation.
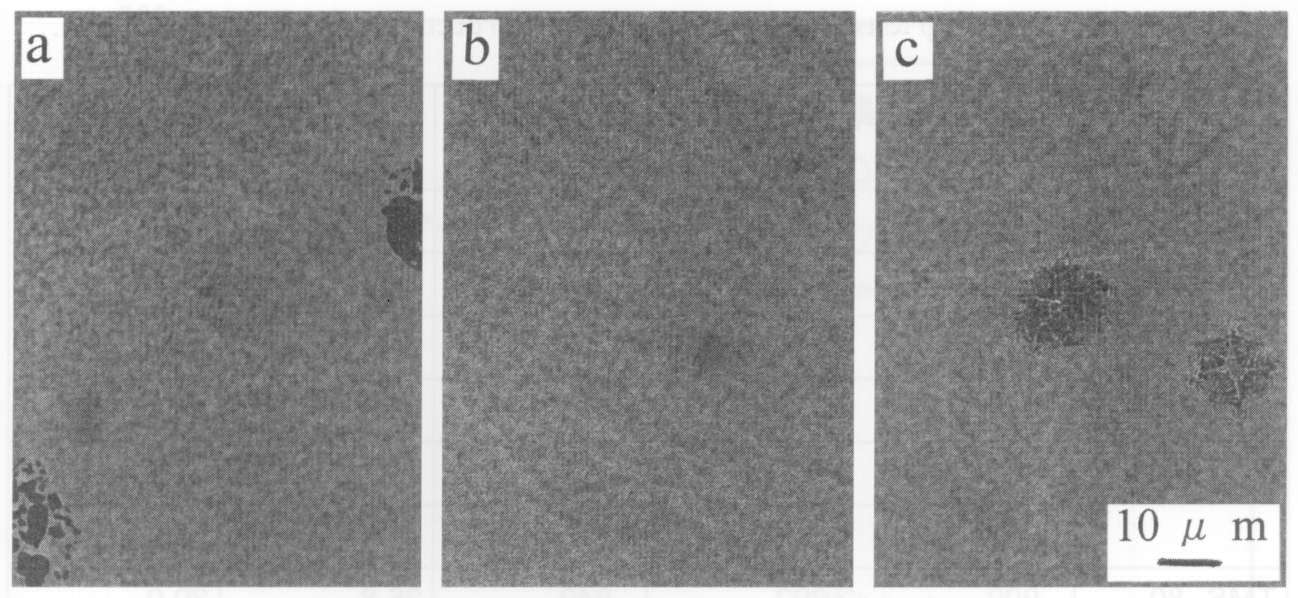
Figure 2 The result of single step solution treatment trials at different temperatures for 2 h with using TMS-75, at 1300$B!n(B(a), 1320$B!n(B(b) and 1360$B!n(B(c).
- Figure 3 shows the microstructures after heating at 1100$B!n(B for 1000 h and air cooled. A small amount of TCP like precipitates were observed in TMS-75 whereas no such a phase is observed in TMS-80. This shows an excellent phase stability achieved by adding 3wt% Ir. Ir has the fcc structure like Ni, and unlike most of alloying elements, W, Ta, Re, etc. According to the binary phase diagram [7] the Ni and Ir make continuous series of solid solutions. This suggests that Ir can play a role of the base metal with Ni. In fact an APFIM work by Murakami and some of the authors [8] showed that 3wt% Ir addition to an alloy TMS-63, which is known to be saturated with other alloying elements, does not cause any third phase precipitation; Ir desolved into the$B&C(Band $B&C(B' phases with a partitioning preference to the$B&C(B phase. The Ir addition thus brings total amount of base elements larger, which makes the alloy thermodynamically more stable. In the present work, Ir was simply added to TMS-75 during melting, which has also changed the structural parameters chosen through the alloy design calculations. A more careful addition of Ir with adjusting other element content using ADP and CVM is planed to improve both the phase stability and creep strength.
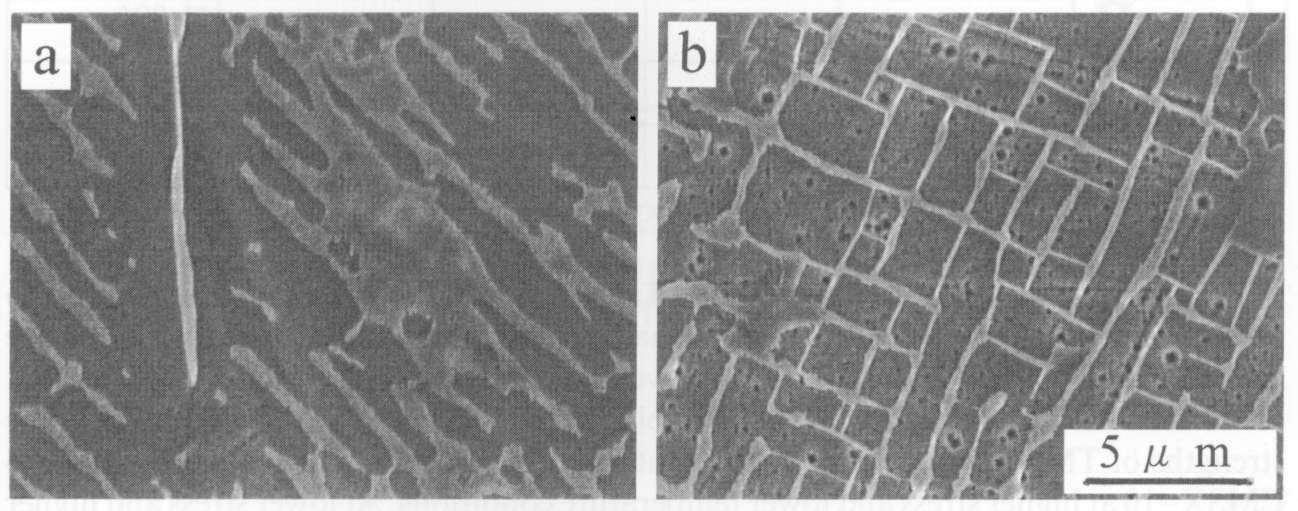
- Figure 3 The microstructures after heating at 1100$B!n(B for 1000 h and air cooled. A small amount of TCP in TMS-75 (a) is disappeared by an Ir addition, TMS-80 (b).
- The results of creep tests are shown in Table 2. TMS-75 and 80 have similar creep rupture properties each other, except for that the elongation and reduction of area are larger in TMS-80. The Larson Miller curves of the alloys are shown in Figure 4 with that of CMSX-10 plotted by the data published by Erickson [1].It is shown that the strengths of TMS-75 and 80 are equivalent to each other. They are also equivalent to CMSX-10 at higher stress and lower temperature conditions. At lower stress and higher temperature conditions TMS-75 and 80 becomes stronger than CMSX-10. Longer term creep tests at high temperatures are scheduled. It is expected that the better phase stability in TMS-75 and especially TMS-80 results in the excellent creep strengths under such longer term creep conditions.
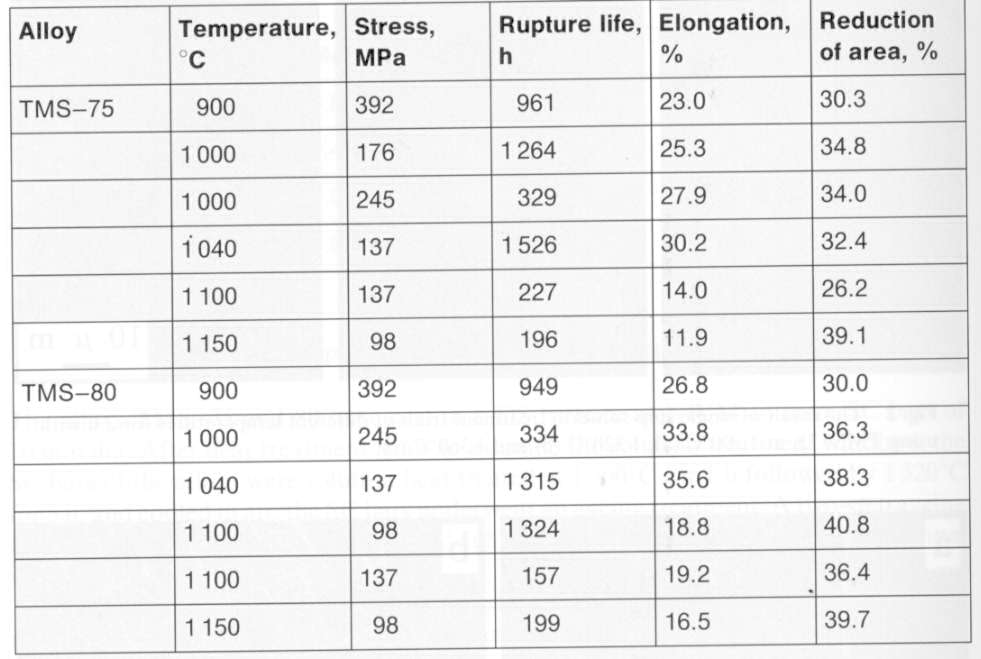
- Table 2 Results of creep tests, showing the excellent creep ductility as well as creep strength.
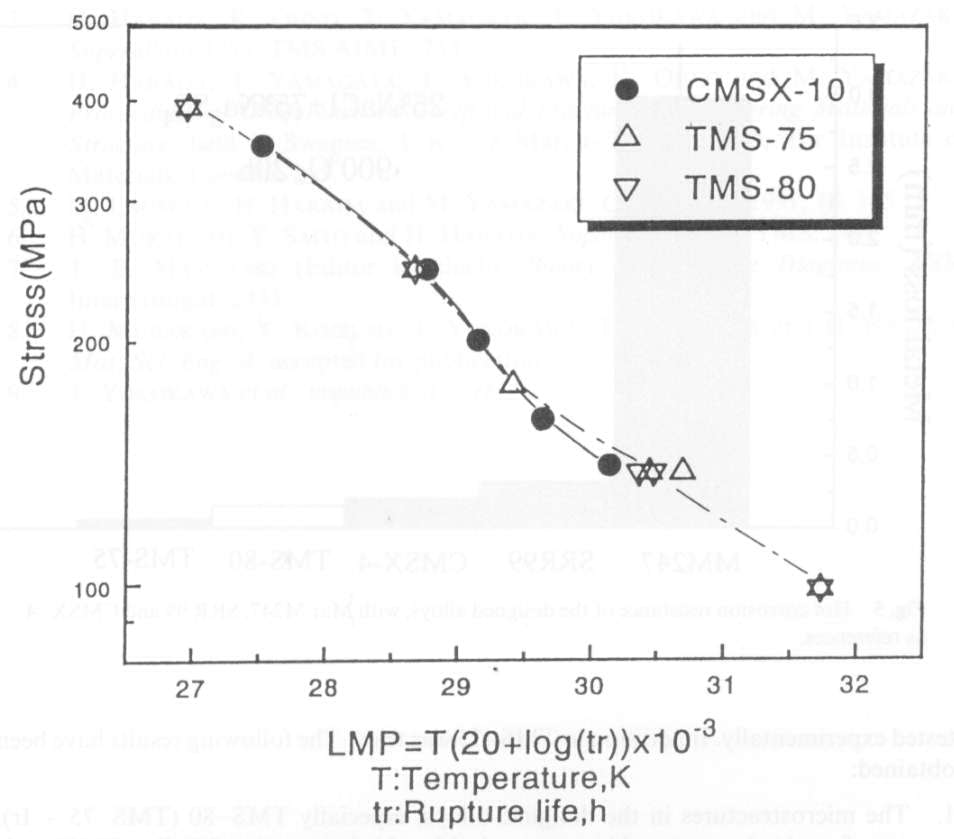
- Figure 4 Creep rupture strengths of designed alloys, TMS-75 and TMS-80, with that of CMSX-10 as a reference.
- The lattice misfit is another important aspect. Due to the low Mo and high Ta contents, the $B&C(B/$B&C(B' lattice misfit in CMSX-10 is calculated by ADP and CVM to be positive at room temperature and also at high temperatures like 1050$B!n(B. In contrast, the lattice misfits in TMS-75 and 80 are both calculated to be negative at high temperatures, which has been verified with using high temperature X-ray diffractometry [9]. The enhancement of rafting by the negative lattice misfit should be another reason for the strengths at higher temperatures in spite of the smaller amount of Re contents.
- The hot corrosion resistance is another important property. Figure 5 is the result of the crucible test using Na2SO4-25%NaCl salt mixture. The designed alloys show excellent hot corrosion resistance which is even better than the second generation superalloy CMSX-4. The hot corrosion resistance may change depending on the testing methods and conditions. Testing at more practical conditions are needed.
- From all the results obtained so far, the TMS-75 and TMS-80 are promising to be alloys with balanced intermediate and high temperature creep strengths, with excellent hot corrosion resistance. The easy heat treatment due to the wide solution treatment windows is another important characteristics for practical applications.
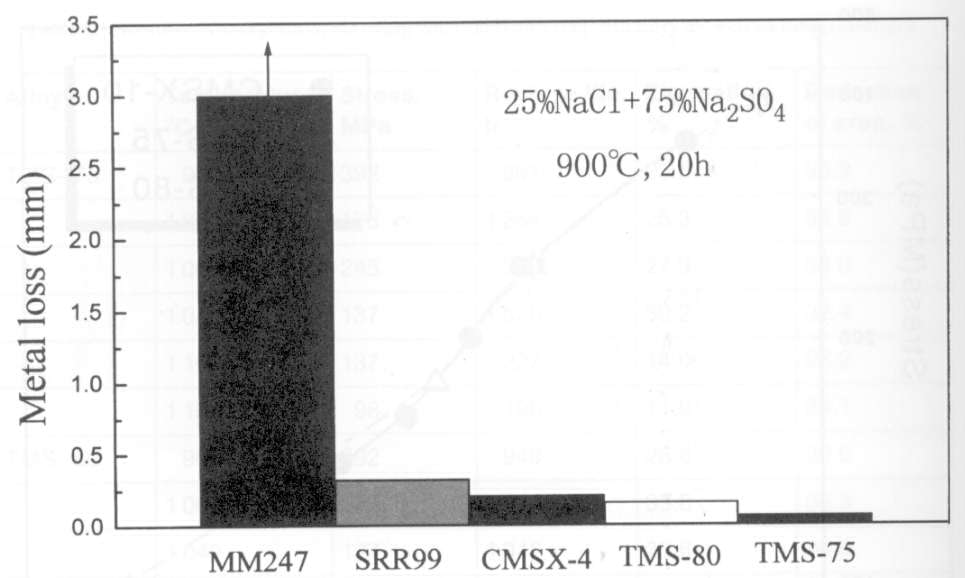
- Figure 5 Hot corrosion resistance of the designed alloys, with Mar M247, SRR 99 and CMSX-4 as references.
CONCLUSIONS
- A single crystal (SC) superalloy, TMS-75, with balanced intermediate and high temperature creep strengths was designed by using NRIM Alloy Design Program (ADP) with help of Cluster Variation Method (CVM) computer program and tested experimentally. Ir addition to TMS-75 was tried. The following results have been obtained;
- 1. The microstructures in the designed alloys, especially TMS-80 (TMS-75+Ir), were found to be more stable compared with a third generation SC alloy CMSX-10.
- 2. The creep strengths of the alloys were equivalent to that of CMSX-10 at higher stress and lower temperature conditions and stronger at lower stress and higher temperature conditions.
- 3. The perfect solution treatment windows of the alloys were confirmed to be about 40$B!n(B wide, which is wide enough to perform one-step solution treatment.
- 4. Thus the TMS-75 and TMS-80 are promising as alloys with balanced intermediate and high temperature creep strengths, with excellent hot corrosion resistance. The easy heat treatment due to the wide solution treatment windows is another important characteristic for possible practical applications for turbine blades and vanes.
REFERENCES
- 1. G.L.Erickson: Superalloys 1996 (TMS), p.35.
- 2. W.S.Walston, K.S.O'Hara, E.W.Ross, T.M.Pollock and W.H.Murphy, Superalloys,1996 (TMS), p.27.
- 3. H.Harada, K.Ohno, T.Yamagata, T.Yokokawa and M.Yamazaki, Superalloys 1988 (TMS AIME), p.733.
- 4. H.Harada, T.Yamagata, T.Yokokawa, K.Ohno and M.Yamazaki, Proceedings of Conference on Creep and Fracture of Engineering Materials and Structure, held in Swansea, U.K., 28 March-2 April, 1990, The Institute of Materials, p.255.
- 5. M.Enomoto, H.Harada and M.Yamazaki, CALPHAD, Vol.15, 1991, p.145.
- 6. H.Murakami, Y.Saito and H.Harada, Superalloys 1996 (TMS), p.249.
- 7. T.B.Massalski(Editor in chief), Binary Alloy Phase Diagrams (ASM International),p.2333.
- 8. H.Murakami, Y.Koizumi, T.Yokokawa, T.Yamagata and H.Harada, Materials Science and Engineering A, accepted for publication.
- 9. T.Yokokawa et al., unpublished work.
High Temperature Materials 21 Project